Attenuated total reflection infrared spectroscopy combined with multivariate data analysis for studying modal composition
Abstract
Quantitative interpretation of results obtained from Attenuated total reflection Fourier transform infrared (ATR FTIR) spectroscopy is difficult and for deeper insight it is necessary to employ various data-processing methods. These methods must be suitable for handling large multidimensional data sets and for exploring the complete spectral information simultaneously. The effective implementation of these multivariate data analysis methods, however, also requires the pre-treatment of data. The pre-processing of raw data helps in the elimination of noise and the enhancement of discriminating features. This study focuses on two commonly-used multivariate methods of analysis: principal component regression (PCR) and partial least squares regression (PLSR); these methods enable the extraction of mineralogical information from infrared spectra. The present study also discusses the various spectral preprocessing methods that are widely used in ATR FTIR spectroscopy. A dataset of natural standards of common rock-forming minerals (calcite, dolomite, quartz, feldspar, muscovite, illite, smectite and kaolinite) and their synthetic mixtures was constructed to build PCR and PLSR models that link the mineralogy of the samples to their respective infrared spectral signatures. Infrared spectra of the samples were recorded from 400 to 4000 cm–1. As a reference, modal composition was also estimated from X-ray diffraction data. The resulting PCR and PLSR models were also tested on synthetic mixtures. The overall conclusion for the constructed 24 models is that, with respect to prediction, PCR and PLSR provide similar results. Different types of spectral treatment have greater impact on the estimated modal composition than the studied multivariate methods. Furthermore, in the models the respective amounts of various minerals were estimated with different uncertainties; this was the result of the difference in the infrared light-absorbing capacity of minerals, overlapping bands and other physical effects.
References
ADAMU, M.B. 2010: Fourier Transform Infrared Spectroscopic Determination of Shale Minerals in Reservoir Rocks. — Nigerian Journal of Basic and Applied Science 18, 6–18. https://doi.org/10.4314/njbas.v18i1.56836
BERTAUX, J., FRÖHLICH, F., ILDEFONSE, P. 1998: Multicomponent analysis of FTIR spectra: quantification of amorphous and crystallized mineral phases in synthetic and natural sediments. — Journal of Sedimentary Research 68, 440–447. https://doi.org/10.1306/d42687cf-2b26-11d7-8648000102c1865d
BRUKER OPTIK 2011: IR Reference Manual. — Opus Spectroscopy Software, Ettlingen, Germany, 120 p.
CHIPERA, S.J. & BISH, D.L. 2001: Baseline studies of The Clay Minerals Society Source Clays: Powder X-ray diffraction analyses. — Clays Clay Miner. 49, 398–409. https://doi.org/10.1346/ccmn.2001.0490507
DE JONG, S. 1993: SIMPLS: An alternative approach to partial least squares regression. Chemom. — Intell. Lab. Syst. 18, 251–263. https://doi.org/10.1016/0169-7439(93)85002-x
DUYCKAERTS, G. 1959: The infrared analysis of solid substances — Analyst 84, 201–214.
EATON, J. W., BATEMAN, D., HAUBERG, S., WEHBRING, R. 2015: GNU Octave version 4.0.0 manual: a high-level interactive language for numerical computations. [http://www.gnu.org/software/octave/doc/interpreter – 2017.06.10.]
GELADI, P. & KOWALSKI, B.R. 1986: Partial least-squares regression: A tutorial. — Analytica Chimica Acta 185, 1–17. https://doi.org/10.1016/0003-2670(86)80028-9
GEMPERLINE, P. 2006: Chapter 4: Principal component analysis. — Practical Guide To Chemometrics, 2nd ed., Boca Raton, CRS Press, Taylor & Francis Group, 552 p. ISBN: 1420018302. https://doi.org/10.1201/9781420018301
GRIFFITHS, P.R. & DE HASETH, J.A. 2007: Chapter 10: Data Processing. — Fourier Transform Infrared Spectrometry, 2nd ed., New York, Wiley-Interscience, 225–250. https://doi.org/10.1002/9780470106310.ch10
HENRY, D.G., WATSON, J.S., JOHN, C.M. 2017: Assessing and calibrating the ATR FTIR approach as a carbonate rock characterization tool. — Sedimentary Geology 347, 36–52. https://doi.org/10.1016/j.sedgeo.2016.07.003
HILLIER, S. 2000: Accurate quantitative analysis of clay and other minerals in sandstones by XRD: comparison of a Rietveld and a reference intensity ratio (RIR) method and the importance of sample preparation. — Clay Miner. 35, 291–302. https://doi.org/10.1180/000985500546666
JORDÁ, J.D., JORDÁN, M.M., IBANCO-CAÑETE, R., MONTERO, M.A., REYES-LABARTA, J.A., SÁNCHEZ, A., CERDÁN, M. 2015: Mineralogical analysis of ceramic tiles by FTIR: a quantitative attempt. — Appl. Clay Sci. 115, 1–8. https://doi.org/10.1016/j.clay.2015.07.005
KLUG, H.P. & ALEXANDER, L.E. 1954: X-ray diffraction procedures. — John Wiley Sons Inc., New York-London-Párizs, 716 p.
KOVÁCS, J & KOVÁCSNÉ SZÉKELY, I. 2006: A minta értelmezési problémái: elmélet és gyakorlat. — Földtani Közlöny 136/1, 139–146.
KOVÁCS I., UDVARDI B., FALUS GY., FÖLDVÁRI M., FANCSIK T., KÓNYA P., BODOR E., MIHÁLY J., NÉMETH CS., CZIRJÁK G., ŐSI A., VARGÁNÉ BARNA ZS., BHATTOA H., SZEKANECZ Z., TURZA S. 2015: Az ATR FTIR spektrometria gyakorlati alkalmazása néhány - elsősorban földtani - esettanulmány bemutatásával. — Földtani Közlöny 145/2, 173–192. http://ojs3.mtak.hu/index.php/foldtanikozlony/article/view/116
MARTENS, H.S. & NAES, T. 1989: Multivariate Calibration. — John Wiley & Sons, New York, 438 p.
MATTESON, A. & HERRON, M.M. 1993: Quantitative mineral analysis by Fourier transform infrared spectroscopy. — SCA Conference Paper 9308, 1–16.
MIRABELLA, F.M. 2002: Principles, Theory, and Practice Of Internal Reflection Spectroscopy. — In: MIRABELLA, F.M. (szerk.): Internal Reflection Spectroscopy: Theory and Applications. — Marcel Dekker, New York, 17–52.
MÜLLER, C.M., PEJCIC, B., ESTEBAN, L., PIANE, C.D., RAVEN, M., MIZAIKOFF, B. 2014: Infrared attenuated total reflectance spectroscopy: an innovative strategy for analyzing mineral components in energy relevant systems. — Sci. Rep 4, 6764. https://doi.org/10.1038/srep06764
NAES, T., ISAKSSON, T., FEARN, T., DAVIES, T. 2002: A user-friendly guide to multivariate calibration and classification. — NIR publications, Chichester, 344 p.
PALAYANGODA, S.S., NGUYEN, Q.P. 2012: An ATR FTIR procedure for quantitative analysis of mineral constituents and kerogen in oil shale. — Oil Shale 29, 344–356. https://doi.org/10.3176/oil.2012.4.05
PLANINSEK, O., PLANINSEK, D., ZEGA, A., BREZNIK, M., SRČIČ, S. 2006: Surface analysis of powder binary mixtures with ATR FTIR spectroscopy. — International Journal of Pharmaceutics 319, 13–19. https://doi.org/10.1016/j.ijpharm.2006.03.048
REIG, F.B., ADELANTADO, J.V.G., MOYA MORENO, M.C.M. 2002: FTIR quantitative analysis of calcium carbonate (calcite) and silica (quartz) mixtures using the constant ratio method. Application to geological samples. — Talanta 58, 811–821. https://doi.org/10.1016/s0039-9140(02)00372-7
RITZ, M., VACULÍKOVÁ, L., PLEVOVÁ, E. 2011: Application of infrared spectroscopy and chemometric methods for the identification of selected minerals. — Acta Geodyn Geomater. 8, 47–58.
SAJÓ, I. 1994: Powder diffraction phase analytical system 1.7. — users guide, Aluterv-FKI Kft., Budapest, 1–81.
SAVITZKY, A. & GOLAY, M.J.E. 1964: Smoothing and differentiation of data by simplified least squares procedures. — Anal Chem. 36, 1627–39. https://doi.org/10.1021/ac60214a047
UDVARDI, B., KOVÁCS, I. J., FANCSIK, T., KÓNYA, P., BÁTHORI, M., STERCEL, F., FALUS, GY., SZALAI, Z. 2016: Effects of Particle Size on the Attenuated Total Reflection Spectrum of Minerals. — Appl. Spectrosc. 61, 283–292. https://doi.org/10.1177/0003702816670914
WASHBURN, K.E. & BIRDWELL, J.E. 2013: Multivariate analysis of ATR FTIR spectra for assessment of oil shale organic geochemical properties. — Org. Geochem 63, 1–7. https://doi.org/10.1016/j.orggeochem.2013.07.007
WENTZELL, P.D. & MONTONO, L.V. 2003: Comparison of principal components regression and partial least squares regression through generic simulations of complex mixtures. — Chemom. Intell. Lab. Syst. 65, 257–279. https://doi.org/10.1016/s0169-7439(02)00138-7
WOLD, S., SJOSTROM, M., ERIKSSON, L. 2001: PLS-regression: a basic tool of chemometrics. — Chemom. Intell. Lab. Syst. 58, 109–130. https://doi.org/10.1016/s0169-7439(01)00155-1
WOODS, B., LENNARD, C., KIRKBRIDGE, K.P., ROBERTSON, J. 2015: Soil examination for a forensic trace evidence laboratory - Part 1: Spectroscopic techniques. — Forensic Science International 245, 187–194. https://doi.org/10.1016/j.forsciint.2014.08.009
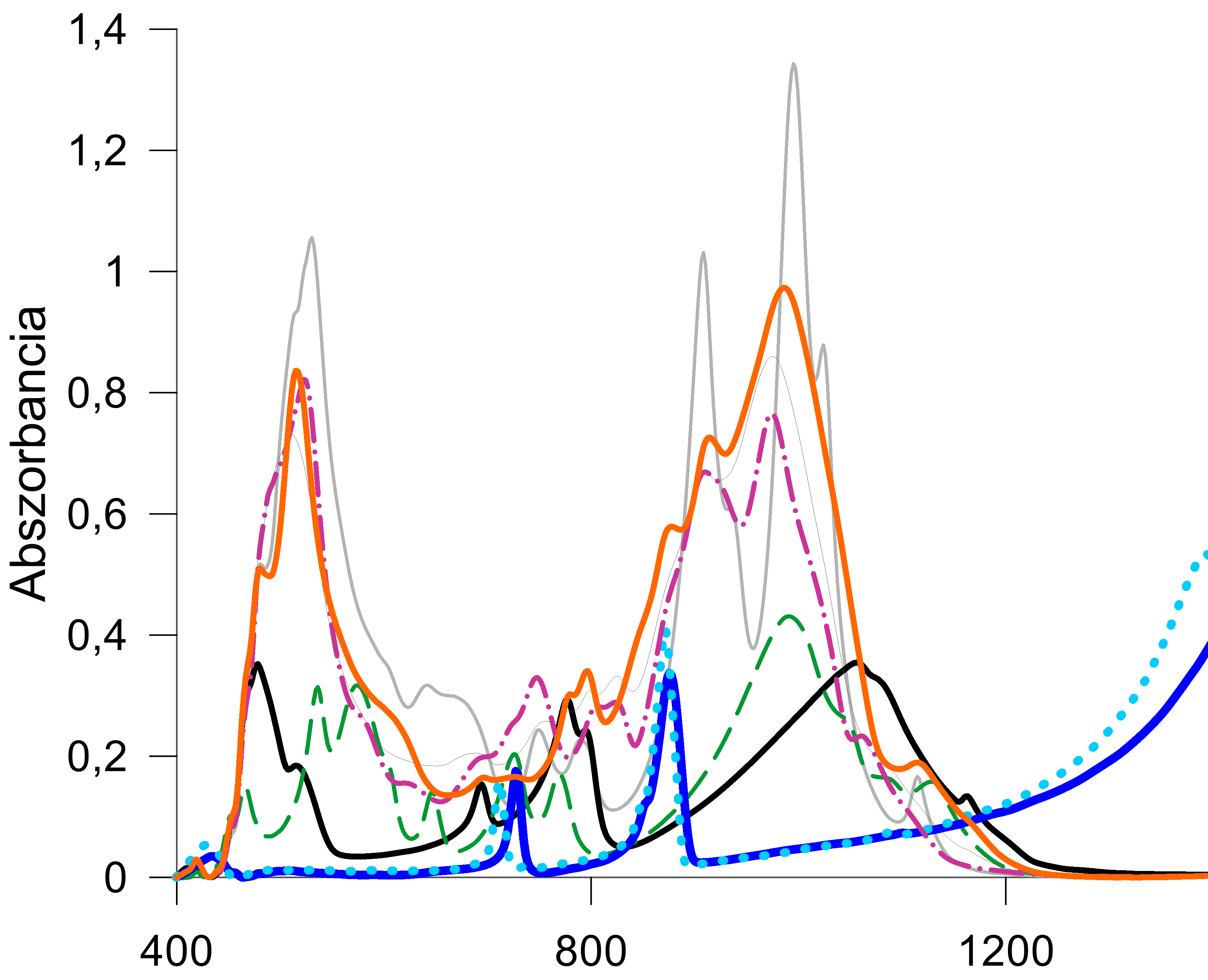